As the demand for clean and sustainable energy continues to rise, wind energy has become a crucial player in the global effort to reduce carbon emissions. However, operating wind turbines in cold climates presents a unique set of challenges. One of the most significant challenges is the formation of ice on turbine blades. Icing on wind turbines can impact their performance, efficiency, and overall reliability, making it essential for the industry to develop effective solutions and work closely with our weather forecasters at Infoplaza to mitigate these issues. In this article we dive deep into the physics and associated risks of ice deposition on wind turbines.
Definition of ice deposition
The deposition of ice on wind turbines is largely defined and moderated by supercooled water droplets in the air (liquid water with temperatures below zero degrees Celsius) which come into contact with the turbine blades and directly freeze upon impact. Obviously, this phenomenon is most common in regions with low temperatures and high humidity. The droplets involved - and to a lesser extent ice particles - usually originate in clouds but can also be formed in specific atmospheric settings which allow for supercooled precipitation to occur. Both can pose a serious threat to the efficiency of wind farms.Water that doesn’t freeze
For water to freeze several conditions need to be met. Within clouds tiny water droplets are suspended in the air, often not larger than a few hundredths of a millimeter. They form when water vapor (gas) condenses onto aerosol particles. These droplets (liquid) are so small and lightweight that they can remain floating in a semi-weightless state in the atmosphere.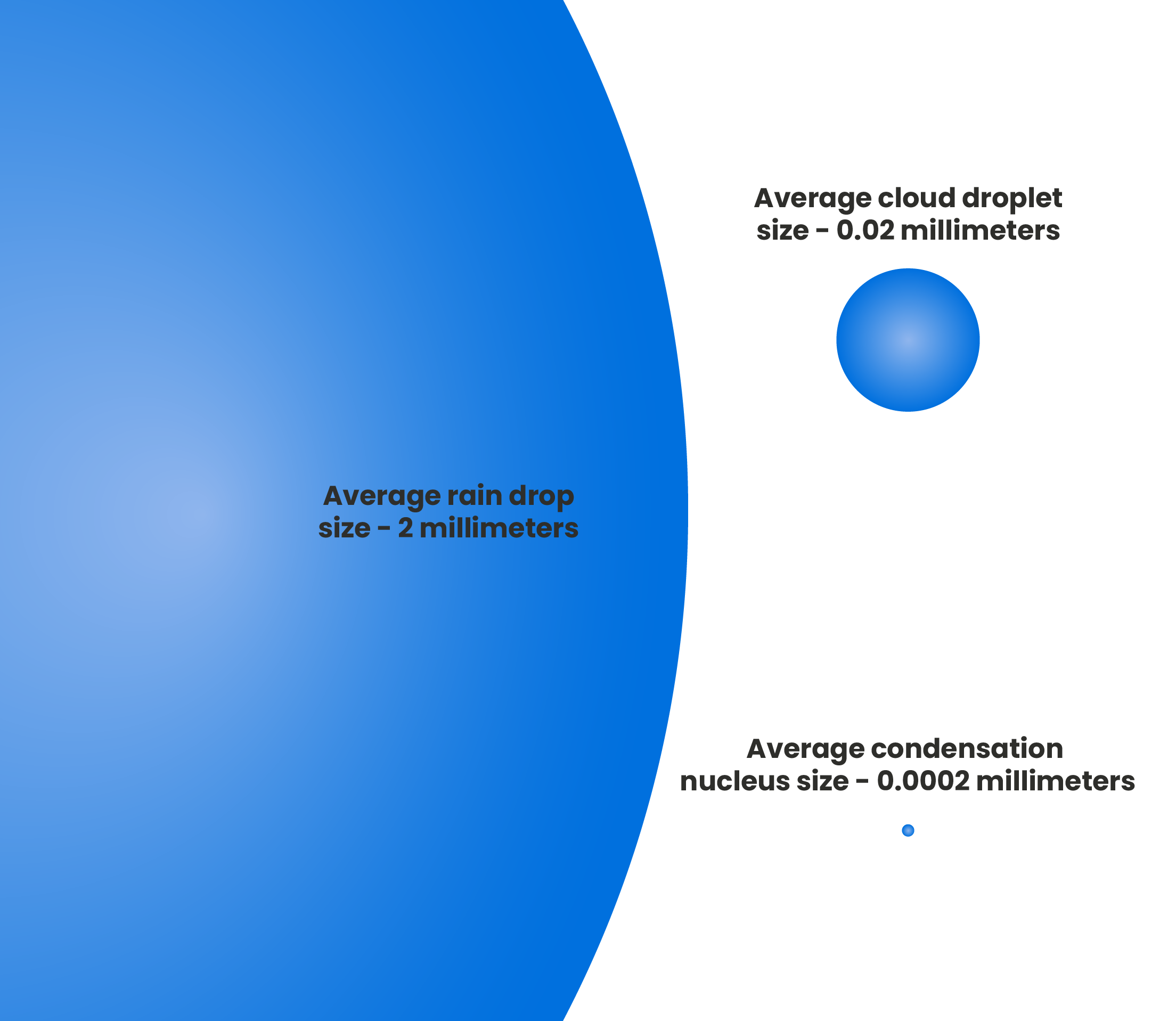
Due to their minimal size the surface tension, when compared to the total volume of the droplet, is predominant. This will prevent a spontaneous freeze-up, even when temperatures drop well below freezing.
Nucleation of water
In order for liquid cloud droplets to transition into a solid icy phase, also called nucleation, we need to: 1) lower the ambient temperature significantly, or 2) add particles that will pay the high ‘surface costs’, or 3) add kinetic energy. To explain all three, we need to look at the critical radius of a droplet and the way this plays a key role in spontaneous nucleation.We now know that without external help surface tension prevents liquid cloud elements from freezing. In absence of ice nuclei (‘helper molecules’) cloud droplets can remain liquid, even well below -30°C! In clinical lab circumstances (with a total absence of ice nuclei and fully clean water) the phase transition from water to ice can even be prevented until temperatures reach a chilling -50°C.
Homogeneous and heterogeneous nucleation
This obviously isn’t the case in our atmosphere. So, when temperatures drop to around-40°C, spontaneous nucleation takes place without significant interference of ice nuclei. This process is called homogeneous nucleation. One can assume that once this temperature is reached almost all cloud elements will consist in solid form.
At higher temperatures ice nuclei come into play. As it takes two or more particles to start ice formation this is called heterogeneous nucleation. The ‘helper molecules’ consist of various chemicals present in the ambient air and resemble an ice crystal due to their partially hexagonal shaped molecule structure. They actually pay the surface costs to start nucleation, which can start from within or along the outer edges of the droplet.
Each compound has its own activation temperature. Note that temperatures displayed below are applicable for nucleation from gas to ice. For liquid water some molecules will activate nucleation already at -3°C, which will lead to a water-ice distribution as shown in figure 2.1.
Figure 2, ice nuclei activation temperatures
Figure 2.1, classic ice-water distribution in the atmosphere
Kinetic energy
Another way of starting spontaneous nucleation is to add (kinetic) energy to supercooled water. This can come from convection, turbulence, and yes, also a turbine blade. All of the previously mentioned nucleation starters have a common effect: they reduce the so-called Gibbs free energy (ΔG) which represents the energy barrier associated with the formation of an ice crystal. This is where the critical radius (r*, the minimum size a droplet must obtain for spontaneous freezing to occur) and ΔG interact; they describe the subtle balance between surface tension and volume energy, determining the state of supercooled droplets at a certain temperature (see figure 3 and 3.1).In other words: when a supercooled water droplet reaches the critical radius, the energy required for the formation of a premature ice crystal becomes lower than the energy required to maintain the liquid state. As a result, the droplet undergoes spontaneous freezing. Heterogeneous nucleation relies on a lowering of ΔG by adding an external force or particle, leading to a quicker freeze-up (fig 3.1).
Figure 3 and 3.1: till r* the surface is predominant. Afterwards, the bulk wins and the phase transition runs freely. Adding energy or an ice nucleus reduces ΔG, enhancing the risk of spontaneous nucleation).
The impact of ice on wind turbines
We now know how and when atmospheric water transforms into ice. This is of high importance to wind farm owners as it is the liquid water content (LWC) of a layer of supercooled clouds that determines the speed and severity of ice deposition; which, on its turn, influences the profile and weight of the turbine blades. Ice crystals are much lighter, don’t attach to blades that easily and are therefore of less importance.
A clean wind turbine efficiently transforms kinetic energy from moving air into mechanical energy (rotation) and eventually into electric energy. When ice accumulates on the turbine blades, they partially lose their ability to do so as the aerodynamic profile of the blades is distorted and the airflow around the blades is disrupted by additional drag. The weight unevenly added by the accumulating ice on the leading edge of the blades can create strong vibrations and imbalances in the turbine's rotating components, causing mechanical stress. This leads to increased wear and tear, potentially impacting the turbine's lifespan.
Also, ice shedding from rotating turbine blades poses safety risks to both on-site personnel and nearby structures. Large chunks of ice falling down can cause damage or injury, necessitating safety measures and precautions.
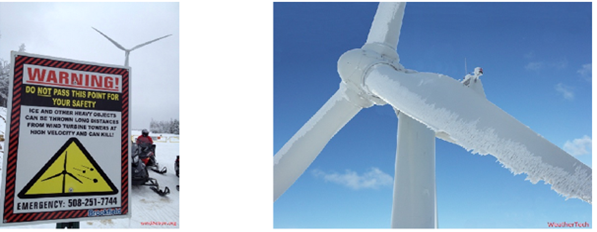
The amount and size of supercooled liquid water droplets dictates the character and severity of ice deposition on turbine blades, possibly reducing the annual power output by roughly 20% when frequent ice events occur. In severe cases short term losses over 50% can be suffered, especially when windspeed is rather low and icing dominates over wind.
This greatly reduces the capacities of the turbine. Ice tends to unevenly accumulate on the leading edge of the blades where supercooled droplets have their direct impact, and to a far lesser extent on the trailing edge due to the curved airflow. Since there is a clear correlation between blade icing and relative velocity, the regions closer to the tip of the blade will accumulate more ice in a shorter period of time, also on the trailing edge at the tip. This results in potentially great imbalances as well as significant drag, grinding the turbine to a halt in extreme cases.
Types of icing
Generally speaking, we can state that the higher LWC the more deposition of ice will occur. 'Trace icing' or 'light icing' occurs when small supercooled liquid elements quickly freeze upon impact; usually in a situation with rather dry air, low LWC (<0.5gr/m³) and (very) low temperatures (<-5°C). This type of icing can even occur when clouds are partially absent, but only at very low temperatures. It typically shows by a rather thin white layer of brittle ice with a lot of air trapped in it, the so-called ‘rime icing’. In case of very dry air and low temperatures accumulation is limited due to constant sublimation.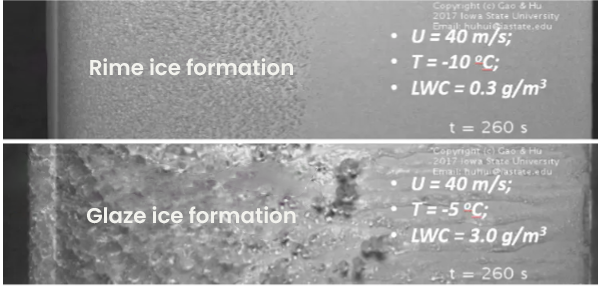
Moderate to severe icing (LWC >1gr/m³) usually appears as a layer of clear dense ice with little or no air pockets, in atmospheric settings with temperatures near or just a few degrees below zero. Obviously, in case of freezing rain this type of icing will prevail and accumulate very rapidly. Due to inertia the large droplets will fully attach to any surface they come in contact with and cause significant weight gains along the entire blade. Supercooled old fields of fog, or forced stable clouds in mountainous regions (like hochnebel) are also notorious for their high LWC and rather large droplets. We call the resulting type of ice deposition glaze or clear icing. This is the worst type of icing and frequently results in significant power loss or even complete blackouts.
In practice, one can also observe mixed forms wedged in between the two previously mentioned types of icing. It usually possesses some of both characteristics and occurs in atmospheric settings with alternating LWC, enabling layers of airy and more solid ice to accumulate on top of each other, usually within the moderate icing classification.